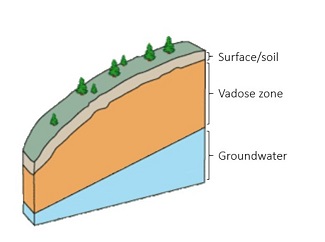
By Adeline Lopez
Researchers funded by the NIEHS Superfund Research Program (SRP) at the University of Arizona (UA) study how hazardous materials move in the environment. By combining mathematical models with laboratory and field studies, the team can better understand factors that cause contaminant cleanup to stall and identify cost-effective solutions to better protect human health.
The research team studies the movement of legacy pollutants, like chlorinated solvents or mine tailings, which remain in the environment long after they have stopped being produced or released. They also study emerging contaminants, like per- and poly-fluoroalkyl substances (PFAS), which are increasingly detected in the environment and have potential to harm human health.
The Problem
When chemicals are spilled or released on land, they move downwards through the soil into a subsurface layer called the vadose zone, and potentially to groundwater. This situation can pose a health risk to humans, who may be exposed through contaminated drinking water.
Every contaminated site has unique soil and water characteristics with different contaminants or mixtures of contaminants. These variable physical and chemical properties influence how contaminants move through the subsurface and dictate the best strategies for cleanup.
Implementing efficient, cost-effective cleanup systems at a hazardous waste site depends on accurately characterizing the type and magnitude of contamination. Risk assessors and site managers must have a thorough understanding of how contaminants are distributed in the subsurface, how they are transported, and how they transform over time.
SRP Solutions
Mark Brusseau, Ph.D., of the UA SRP Center studies the physical, chemical, and biological processes that control contaminant movement through the subsurface. For over 30 years, Brusseau and colleagues have focused on understanding the different factors that control the transport of chlorinated solvents, metals and mine tailings, and others, and therefore how much of the contaminant can reach groundwater.
"We need to know where contaminants are, what their concentrations are, and how fast they move," said Brusseau. "We can use that information to make predictions over time or about how different cleanup approaches will behave."
According to Brusseau, SRP's sustained support has been essential for moving this work forward.
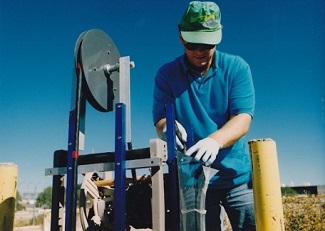
Brusseau works at a field site in 1995.
(Photo courtesy of Mark Brusseau)
"SRP's support for basic science is unique among funding agencies," he said. "It has allowed us to understand these processes more deeply, and we've been able to leverage SRP funding to win or supplement other grants to apply our research in the field. Combining these factors with SRP's focus on research translation and working across disciplines has allowed us to apply this research and see real benefits for impacted communities."
Together, Brusseau and his team are making strides to improve existing cleanup technologies while providing the basis for designing more efficient and effective solutions.
Multidisciplinary Approach
According to Brusseau, including experts with diverse expertise, such as hydrologists, environmental engineers, and environmental chemists is a critical component of their success.
"We utilize a lot of different skill sets to help tackle complex, real-world problems," he said. "All these disciplines are interconnected, and SRP has been a leader in recognizing the value of multidisciplinary research to solve pressing environmental health questions."
Together, their team integrates advanced mathematical models, lab, and field studies to better capture the complexities of the entire system under realistic conditions.

"Translating research from models to the lab and to the field is not a linear process," explained Brusseau. "There are lots of iterative feedback loops where each component informs and enhances the others."
For example, he explained how leveraging mathematical models provides a cost-effective strategy to tackle the nearly infinite combinations of realistic site variables over time. The team can test various scenarios or simulations and use models to explain observations in the field or make better predictions.
Collaboration Fuels Discoveries and Cost Savings
Brusseau's early work focused on creating mathematical models to better represent the sorption of contaminants as they move through pores in the subsurface and predicting the spread of contaminants. Much of his work centered on studying chlorinated solvents, which are commonly found at Superfund sites and are relatively stable in the environment, posing unique challenges for cleanup.
Continuing this work, Brusseau and his team collaborated with diverse stakeholders to characterize contaminated sites, evaluate traditional cleanup approaches, and understand why they stall.
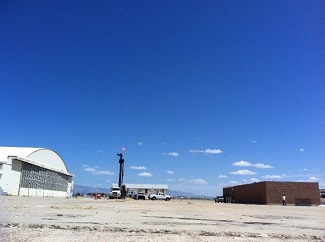
Brusseau and team collect core samples at a field site in 2012.
(Photo courtesy of Mark Brusseau)
"Being able to collaborate and work on an actual Superfund site and other hazardous waste sites allowed us to make connections between what we were seeing in the lab or with models and what was happening in the field," Brusseau explained. "Combining this information helped us figure out why different cleanup strategies weren't working."
For example, in collaboration with the U.S. Air Force, Ratheon Corp, local consulting firms, and federal and state regulatory agencies, the team combined field experiments, modeling, and pilot studies that revealed important information about factors controlling water flow and the success of pump-and-treat cleanup systems. They also developed a model to evaluate the long-term operational performance of a pump-and-treat facility.
Working with consulting companies, Brusseau analyzed the amount of contaminant that discharged into the environment to assess the effectiveness of in situ chemical oxidation treatment, and whether it was still needed. He also identified new strategies to enhance site cleanup using this method.
Brusseau collaborated with researchers at the Pacific Northwest National Laboratory to develop an approach to evaluate changes in contaminant concentrations during soil vapor extraction (SVE) to estimate time frames for effective treatment. Their discoveries about SVE performance support decision making for optimizing or terminating SVE operation, and demonstrated the effectiveness of an approach for evaluating SVE cleanup at three different sites.
By combining data collected at the Department of Energy's Hanford Site in Washington state and conducting follow-up tests at a national priority site in Tucson, Arizona, they were able to provide more information about the amount of work needed to remove contaminants at the Hanford Site, resulting in projected cost savings of over $6 million.
Developing Tools
Brusseau developed the Integrated Contaminant Elution and Tracer Test Toolkit (ICET), which uses specialized tracer tests to study contaminant movement. Tracers have known properties and behaviors, which can be used to follow the behavior of different contaminants, such as sorption or diffusion.

Brusseau's team uses tracers to determine which processes are involved in contaminant transport and to evaluate cleanup performance. ICET characterizes contaminant mass transfer, stalls, and mass removal processes, which can improve the accuracy of risk assessments and can inform decision making about cleanup strategies.
Brusseau and his team combined detailed characterization using ICET tests with lab experiments, mathematical modeling, and pilot tests to understand and improve the effectiveness of cleanup at the Tucson International Airport Area Superfund site.
The information obtained from the ICET tests played an important role in identifying the specific processes contributing to contaminant behavior and persistence and led to the implementation of additional strategies to achieve cleanup goals.
Tackling Emerging Contaminants
"In addition to our current SRP-funded work on movement of metals and mine tailings in the environment, we were uniquely positioned to build on decades of previous SRP-funded research to lead the field in studying PFAS transport," he said.
PFAS are a large group of persistent compounds found in, for example, aqueous film-forming foams, which have been used for fire suppression at airports, industrial facilities, and military sites, and in everyday products.
Brusseau and collaborators revealed important information about factors controlling PFAS migration in vadose zones, specifically adsorption at the air-water interface, for perfluorooctanoic acid and perfluorooctanesulfonic acid. They also examined the movement of PFAS in soil and developed a comprehensive retention model for PFAS transport in the subsurface.
"Based on our earlier work funded by the SRP, we predicted that PFAS would tend to adsorb, or stick, at the air-water interface," said Brusseau. "We confirmed those predictions, but it's important to remember that PFAS are a large group of compounds with a mix of unique properties that control their movement in the subsurface and groundwater."
Using 42 different PFAS, the team evaluated the influence of chemical structure on PFAS adsorption at different interfaces. They used a tool called quantitative-structure/property-relationship analysis to predict their behavior based on the size of the PFAS compound. This provides a useful tool for understanding how different PFAS move through the environment, designing better approaches to cleanup PFAS, and helping risk assessors make better decisions to protect health.