Features
Bioremediation
NIEHS Superfund Research Program (SRP) individual research projects were in the spotlight during the Spring 2022 SRP Progress in Research Webinar series. These projects received funding in 2020 in response to a call for applications for work that combines materials science and engineering approaches with bioremediation science. Bioremediation is a cost-effective, energy-efficient approach that uses bacteria, fungi, and plants to break down and remove hazardous substances from the environment.This feature highlights how these novel strategies to clean up the environment and improve public health.
Cleaning Up Emerging ContaminantsResearchers are devising innovative remediation strategies to remove per- and polyfluoroalkyl substances (PFAS), a large class of chemicals used in a variety of industrial and consumer products, and other halogenated contaminants from the environment.
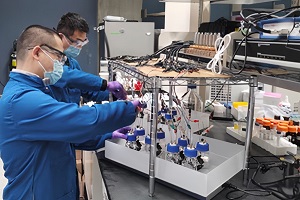
Known as forever chemicals, PFAS are hard to break down because of the strong chemical bonds that hold the molecules together. To address this challenge, grantees are using nanotechnology and other materials science to break down PFAS into smaller, non-toxic molecules that can be easily removed from the environment.
- For example, researchers led by Yujie Men, Ph.D., of the University of California (UC), Riverside, and Chong Liu, Ph.D., of UC Los Angeles, are using nanomaterials powered by solar electricity to break down halogenated contaminants, such as PFAS and a chemical called 1,4-dioxane in groundwater. Their approach uses microwire array electrodes - devices that contain thousands of small electrodes - to allow biological and electrochemical remediation to occur at the same time. Electrochemical remediation relies on the movement of electrons to break down contaminants.
The scientists are also using advanced analytical tools to understand how solar electricity can allow their system to simultaneously degrade halogenated compounds and other co-contaminants faster and more completely. -
Jaffé and Koel are generating cultures of a bacteria called Acidimicrobium sp. strain A6, which can break PFAS bonds. (Photo courtesy of Peter Jaffé)
The nanoparticles are coated with polyacrylic acids, biodegradable materials commonly used in food packaging and diapers to retain water. These acids speed up nanoparticle movement so they reach bacteria more quickly and create conditions favorable to PFAS degradation. To test the effectiveness of their technology, the scientists will use sediment samples collected from a PFAS-contaminated site. -
Aga (left) and team, including postdoctoral researcher Utsav Thapa, Ph.D., (right), are analyzing every step of the reaction to make sure it does not generate toxic byproducts. (Photo courtesy of Diana Aga)
Different PFAS compounds have different molecular structures, so the team is using molecular modeling to identify enzymes from bacteria likely to degrade structurally diverse PFAS. To start, they are focusing on smaller molecules that make up PFAS and will analyze larger PFAS molecules once more data is available.
Optimizing Sorbents for Chlorinated Compounds
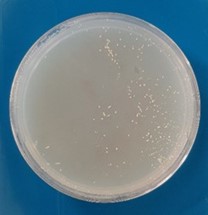
Using advanced sorbents - materials that bind other substances to their surface - and bacteria, researchers are improving techniques to sustainably clean up chlorinated contaminants.
- Youneng Tang, Ph.D., of Florida State University and Yuexiao Shen, Ph.D., of Texas Tech University are developing a new technology to clean up groundwater contaminated with mixtures of 1,4 dioxane and chemicals called volatile organic contaminants (CVOCs).
Historically, bioremediation of CVOC and 1,4-dioxane mixtures has not been possible because low concentrations of 1,4-dioxane are difficult to degrade, and different environmental conditions are required to metabolize 1,4-dioxane and CVOCs. In mixtures, CVOCs can also inhibit the degradation of 1,4-dioxane. To overcome these challenges, the researchers are designing sorbents made up of repeating rings of sugar molecules , called cyclodextrins, to selectively absorb contaminants and promote the growth of biofilms - communities of microorganisms that stick to a surface - that can break down contaminants.
Using a combination of computational and mechanistic studies, the researchers are characterizing and optimizing sorbents for CVOCs and 1,4-dioxane while determining the key microorganisms responsible for degrading those contaminants. So far, their sorbents have shown strong affinity for CVOCs in mixture, but not for 1,4-dioxane, a limitation they are working to address. They also characterized a bacterium that could be a good fit for degrading 1,4-dioxane at low concentrations. -
Hydrogel beads being fabricated in the lab. (Image courtesy of Lewis Semprini)
Their approach involves encapsulating bacteria in tiny spheres called hydrogel beads , along with a compound that stimulates their growth. That compound also generates an enzyme that promotes bacterial degradation of contaminants. The team is currently focused on improving longevity of the beads and the activity of bacteria inside. Initial laboratory and field studies, as well as computer modeling, could inform long-term bioremediation solutions for a broad range of contaminants -
Bacteria attached to a biochar particle. (Image courtesy of Timothy Mattes)
Naturally occurring bacteria can break down these contaminants in the field but are often limited in their ability to finish the job, potentially leaving harmful degradation products behind. Building off initial findings that biochar derived from poplar trees helps bacteria fully degrade halogenated pollutants, the team will be developing biochar-like polymers that can be highly customized. They hope to influence the growth of microbial communities that can break down specific halogenated contaminants within mixtures while simultaneously taking up other toxic compounds. -
The team is developing a variety of carbon-based sorbents to identify physical and chemical structures that can inform bioremediation strategies. (Photo courtesy of Upal Ghosh)
Building off previous work, which demonstrated that bacteria have the capability to degrade PCBs in the presence of activated carbon, the team will produce carbon-based sorbents to enhance biodegradation. They are evaluating the mechanisms of interaction between sorbent surfaces and contaminant-degrading bacteria to improve the degradation process. The team plans to take their research from the lab to the field by testing their technology at contaminated sites.
Using Plants and Fungi to Clean Up the Environment
Researchers are also using advances in materials science to improve how plants and fungi remove hazardous substances from soil and water.
-
Hemp growing on a PFAS-contaminated field site. (Photo courtesy of Vasilis Vasiliou)
The researchers are studying how the length of PFAS molecules - legacy PFAS are longer, emerging PFAS are shorter - affect their uptake by plants. They are testing their nanomaterials using a mixture of two legacy PFAS, perfluorooctane sulfonic acid and perfluorooctanoic acid, and two new PFAS, perfluorobutane sulfonic acid and GenX. -
The researchers are conducting laboratory studies using Cambre abyssinica, a plant known for its rapid growth and high biomass. (Photo courtesy of Om Parkash Dhanker)
The team is altering the expression of certain genes so that the plant will store arsenic above ground in its tissues instead of its roots. By adding nanosulfur, they aim to improve the plant's growth and its ability to convert arsenic into a form less toxic to the plant. The scientists plan to compare the bioremediation efficiency of their engineered plants to controls in contaminated soils. Insights from this study will inform bioremediation approaches to removing arsenic from croplands and contaminated sites. - Fungi play an important role in bioremediation because they can decompose carbon and metabolize organic matter similar in structure to environmental contaminants. However, several factors limit their bioremediation efficiency, including slow growth, bacterial competition, and nutrient requirements.
In laboratory studies, the team observed that their NFF can sustain fungal growth on a petri dish without external nutrient sources. (Photo courtesy Susie Dai)
to Top